Open Source Medicine
This page explores the application of automated information technology to healthcare, looking at how artificial intelligence can help automate healthcare and open collaboration can provide a more effective translation of knowledge into actual patient care.
The medical systems now in existence are fraught with problems. The enormous amounts of data gathered remains disconnected and scattered. Doctors are completely overwhelmed. Cancer and heart disease are on the rise in rich countries, while in poor countries infectious diseases like AIDS storm on unabated. Much research (especially pharmaceutical) is done by private companies who take decades to develop drugs, and are more interested in baldness than in malaria. An unnecessary scarcity of proper healthcare lifts it out of the reach of the poor. A single solution can simultaneously make healthcare provided to the well-off more effective and make healthcare accessible to the poor. This solution is information-rich, open medicine. By properly integrating information revolution technology into our healthcare system, we can provide, at little or no cost to the user, a healthcare system more effective than anything we have imagined. We can switch from a system where medical treatment depends on scarce expertise and drugs to a system where it depends on ubiquitous information.
Information-rich medicine
There are three elements in an information-rich medical system, all of which are greatly empowered by information technology: information must be gathered, processed, and communicated. These are explored in greater detail below. There are compelling reasons for setting up this information infrastructure according to open-source principles, where the platform and its content are built collaboratively, rather than being in the hands of a particular institution.
Gathering information
- Genomics - It now costs only a few thousand dollars to sequence a genome, whereas the Human Genome Project in the nineties took $300 million. If this trend continues, then by 2013 or 2014, getting your genome sequenced will be as cheap as getting your eyesight tested. This data is very valuable to understanding what diseases you are at risk of, whether you will respond to a certain drug, and what cancers you may be susceptible to. The Cancer Genome Atlas
is mapping the genetic basis of cancer. Once this is complete, and cheap genome sequencing is available, we will be able to characterize cancers much more accurately than by body part.
- Proteomics - Mass spectrometry can now get many gigabytes of information out of a single drop of blood. The resolution of the most sophisticated devices is such that every atom can be seen. With genomics and proteomics we can understand and diagnose disease based on the actual molecular processes involved - a much more detailed picture than just looking at symptoms.
- Microfluidics
- Instead of mixing vials of blood with test-tubes of reagents to perform tests, we can now mix mere microliters of each fluid together, to provide the same results on a device the size of a credit card or smaller, costing thousands of times less and taking seconds rather than hours. The biggest epidemic of our time - HIV/AIDS - can now be diagnosed by a chip the size of an iPhone, costing much less money and time than other tests [2]. This technology will allow for a much more effective program to test entire populations for HIV, allowing everyone to know their HIV status, and this will help stop the spread of the virus.
- Medical imaging - Medical scans, such as PET scans, CT scans and MRIs, have recently achieved extraordinary levels of resolution. The resolution is doubling every year and shows no signs of stopping; it is expected to increase a million-fold in the next 20 years. This video demonstrates just how much detail it is possible to see when several kinds of high-resolution 3D scans of the body are put together.
- Electronic medical records - By moving medical records from paper to digital formats, we can share them more easily, and allow them to be accessed around the world, rather than being held in one hospital. This includes such important clinical data as medical history, family history and environmental exposures.
- Sensors - Sensor technology too has been dramatically miniaturized in recent years. There are now wearable heart rate monitors, blood glucose monitors, pedometers and blood pressure cuffs that can gather data 24 hours a day and log it on a computer or smartphone. In the next years, they are expected to shrink further and be implanted in clothes or even inside the body. One advantage of these devices is that they monitor constantly, rather than providing a one-off reading, as when you go to a doctor for a check-up.
- Research - The number of research papers is growing rapidly. Our scientific understanding of disease has grown explosively in the past decade, but there has been no change in the rate of adoption of this data. The answer is to digitize research papers and share them openly on the Internet. There now exists software that can read and understand natural language quite well; if this is applied to research papers, it will be able to use them to inform its decisions.
These are the enabling tools of information-rich medicine. Using them in concert, you can build up a highly-detailed computerized set of information about what is going on with your body. Of course, to use the data effectively, it must be processed.
Processing information
This river of raw data would do a doctor no good; it would simply be overwhelming. This is where the pattern-recognition abilities of artificial intelligence are required. In the same way that artificial intelligence can recognize patterns showing a coming upswing in a stock market, it can recognize patterns in a heart rhythm indicating a coming heart attack. By applying A.I. to the data generated, we can turn raw data into clinically-relevant knowledge. This is already common practice in hospitals, where A.I. is routinely used for tasks like analyzing electrocardiograms.
But can modern artificial intelligence really diagnose disease as effectively as human doctors? The surprising answer is that even mid-1970s artificial intelligence could. In the 1970s, an A.I. called MYCIN proved itself able to perform medical diagnosis as well as, or better than, human doctors.
A patient can even do much of this processing on his or her smartphone. Smartphones can do blood glucose analysis, reconstruct ultrasound images and read and diagnose ECGs. Tasks demanding more computing power will require distributed cloud computing.
One application of this is rational drug design, where A.I. considers the molecular basis of disease and computes the exact molecular intervention that is needed. This is a vast improvement on the old way of designing drugs based on chemical families.
Even if the sophisticated information-gathering methods just described are not fully exploited, using existing artificial intelligence to process clinical information can greatly improve clinical outcomes. Imagine for a moment that if you walk into a clinic, modern information technologies whirr into action and pull from a distributed global network your medical record and family history. You describe your symptoms to the doctor, who inputs them into a handheld computer. The computer processes the symptoms and cross-references them with a database of known diseases. It compares all similarly-presenting cases – no matter where in the world those other cases happened. It uses all this information to create a list of possible diagnoses, then uses optimized and self-improving artificial intelligence algorithms to calculate the likelihood of each one, based on your medical history, race, age, sex and so forth. The computer displays a list of possible conditions and possible courses of action, including treatments or further testing. If a scan is done, say an X-ray, the computer can identify patterns in it and use this to inform its diagnosis. Such a system would never overlook a piece of data such as a drug interaction or allergy, and could intelligently update itself based on the entirety of mankind's medical knowledge. Add to this proteomics, microfluidics, genomics, always-on sensors and high-resolution scanning and the A.I. would become the most powerful healing technology ever created, yet also the cheapest and most accessible.
Communicating information
Because so many different sources of clinical information are available, there must be an effective way of gathering them together, so that A.I. can make a fully-informed decision based on all the data. The clinical data must be communicated to the doctor, to the patient, and into databases of medical information.
All doctors have some gaps in their knowledge. Their job is so complex, and they have so many things to keep track of, that they often simply forget simple things. Human error in healthcare causes hundreds of thousands of deaths. As explained in this presentation, computer programs that communicate reminders of evidence-based best practices to the point of care can overcome this problem.
The ubiquity of digital communications in the modern world offers an exciting opportunity to deliver healthcare information exactly where and when it is needed. Mobile phones, social media and other digital devices can deliver the advice and guidance that a doctor would, but whereas a doctor only communicates with the patient when he is in the clinic, a mobile phone could, for example, give reminders to take pills at the right time.
Artificial intelligence improves itself by studying databases, and the larger the database, the better it gets. Therefore there must be some way of collating results from large numbers of people, to optimize the A.I. systems. If high-quality healthcare is going to be made available to all human beings, information-rich medicine must therefore include a cloud computing database and clinical outcomes must be communicated to this database. This is explored further in the section on open medicine below.
The results of information-rich medicine
Information-rich medicine has several noteworthy features -
- It is good at detecting diseases before they happen. Always-on sensors, genomic risk profile and other data gathered can identify people who are at high risk of disease, or identify the early signs of a disease before noticeable symptoms arise. This allows for cancer to be detected early on, and for many diseases to be avoided entirely with simple preventive measures. Diabetes could be almost eliminated with such an approach. The biggest killer of all - cardiovascular disease - could be controlled very well by always-on sensors that detect heart events before they happen so that preventive action can be taken[3]. Stroke can be predicted quite well from lifestyle factors, blood pressure and MRI scans [4] and is, to a large extent, preventable[5][6], so if people found to be at high risk could be warned with digital communications, a large fraction of strokes could be prevented.
- It allows personalized treatments. Because there is so much information about you personally, drugs need not be prescribed on the basis that they worked for a majority of people in a trial. Instead, you get the treatment most appropriate to your personal biochemistry. This is especially promising for cancer, which is heterogeneous in a way that infectious diseases are not.
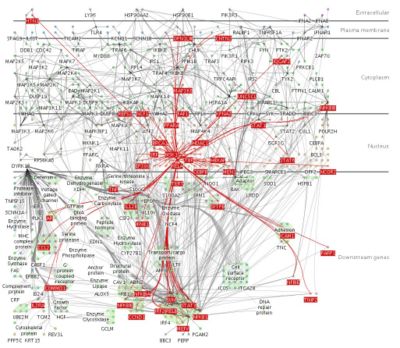
- It lends itself readily to telemedicine. Rather than having to visit a hospital, diagnosis, prescription and monitoring can be done on the basis of sensor readings, videoconferencing and other forms of electronic communications. There are exceptions, such as where a doctor must feel the patient's body, but the majority of cases can be dealt with from home. Surgery is one case where physical contact between the doctor and patient seems necessary. However, this is not necessarily so. Telesurgery
, where a surgeon in one part of the world controls a surgical robot in another, has been performed many times. Rather than get to a specialist surgeon, you would just need to get to the nearest surgical robot, or get it to come to you.
- It considers the whole biological system
, rather than just the disease. It can look at the interactions of environment, nutrition, activity, drugs, sleep and other interventions and how they might tip the complex dance of molecules into a healthier state. The prescriptions might not attack the disease directly, but may be something as simple as a change in diet, which in turn changes the body's production of a certain hormone, which in turn alters the immune system, which in turn attacks the disease. A strength of artificial intelligence is its ability to look at complex systems of data and descry connections that would not be obvious to human intelligence. This allows it, for example, to find patterns in stock market statistics. The same technology applied to biological systems could find interventions that no person would think of.
Open medicine
An information-rich medicine system will be most effective if it is open-source in three ways, which we can call open access, open data and open development. Open access here means that anyone is free to access it free of charge (as with any free website, such as YouTube). Open data means that everyone's data is added to the store, in order to improve the database for the benefit of all (as with Wikipedia). Open development means that motivated people put work into developing the tools.
Open access
All the informatics just described can (and, morally speaking, should) be free to access. Information-rich medicine is a digital product; the value is no longer in the skills of a particular physician, or in a specially-formulated molecule, but in the information. Unless there are institutional restrictions, all digital products can be free and abundant, as happened when music became digital. The cost of storing and transmitting data is tiny, compared to the costs of highly-skilled doctors with complex infrastructure, so there is really no reason medical A.I. should not be made available too all.
For further discussion of open medical A.I., see these links -
- http://www.phoenix.tc-ieee.org/016_Clinical_Care_Support_System/Open_CIG_9_19_sanitized.ppt
- AI in medicine page at Open Clinical
- Presentation of EgaDSS making a very convincing case for the need for open-source medical A.I.
Open data
Rather than being stored in the private databases of a company or healthcare provider, it would be best if people's clinical data (suitably anonymized) were added to a global database. This would give the artificial intelligences more to learn from, allowing them to come to better understandings of disease. If the data is crowdsourced in this way, much more data and feedback can be gathered into the system than if data flows are restricted by secrecy and intellectual property. Compare this to the large pharmaceutical and medical companies of today, whose data is kept in-house and carefully guarded, or to paper charts kept in hospitals and referred to by only one doctor; there is no way such a system can amass anything near the same quantity of data as an open-source initiative.
Mass sharing of data is possible only if free and open-source software is used; if different doctors are using different private software, their records will be incompatible, but if they are all using a common system, their records can inform one another.
Where data is held privately by separate parties, the formats are incompatible and the opportunity to integrate is lost. Of 70 commercial attempts to bring Clinical Decision Support Systems (medical A.I.) into hospitals, none have really caught on, because the task of generating a database of medical knowledge large enough to be really useful has proven to be outside the capacity of any one company [7], in exactly the same way as creating an encyclopedia as big as Wikipedia proved beyond private groups. Any database of clinical information becomes drastically limited as soon as it is made private. The only way to create such a system is as free and open-source software — allow anyone to contribute to the database, get college students, government departments and data entry companies to pile the results of medical research into the database, get software programmers to improve the AI, get clinicians to put the system into practise and record clinical outcomes, and you soon have a database approaching the ideal described above. It is achievable within a few years, but only with global open collaboration. If Wikipedians could do it for fun, then surely the world's medical forces can do it to save lives and unburden themselves.
Open development
- Open-source clinical hardware - There are several pieces of medical equipment whose design is released under open source hardware licenses. They are listed here.
- It will have been noted that some of the information-gathering tools mentioned above require access to scanning machines that are currently very expensive. Though these are becoming smaller and cheaper as the technology improves, by open-sourcing the hardware, we can manufacture them at a fraction the current cost.
- There is a need for cheap, replicable, robust, open-source versions of all medical equipment. This project is well under way: hospitals like the Heart Institute of the Caribbean have developed low-cost versions of medical technologies and are training others to replicate them.
- The benefits of decoupling intellectual property restrictions from medical technologies can be seen with generic drugs. When the patent expires on a drug (making it a sort of 'open-source drug'), the drug typically becomes ten times cheaper.
- Open-source clinical software - There are many very successful pieces of open-source medical software, from electronic medical records to image-recognition software. See Wikipedia's list of open source healthcare software
.
- Open Source Scientific Research applied to medicine - Using open-source principles for medicine may sound a little scary, but anything developed openly can be as rigorously tested as proprietary developments. Potentially, testing can be even more rigorous, as openness will allow more 'eyeballs' to clearly see progression of the project, to contribute knowledge and ideas, and provide additional checking.
- Open collaborative drug development is being pursued by several groups. The Open Source Drug Discovery Network is a large collaborative project that, among other achievements, wat the first group to successfully map the genome of tuberculosis. Many for-profit firms are unwilling to research diseases if they cannot see a lucrative market for it or if the risk of unsuccessful research is too high. Open-source research can tackle diseases which are not financially lucrative, including diseases of poverty like leprosy. Pink Army is a patient-controlled co-op that develops individualized drugs for treating breast cancer.
- Open-source science is emerging as the dominant way of doing biology. A huge amount of information about how biological systems, including the human body, work is compiled in open access sites. Open WetWare is a massive hub of open collaboration among biologists and biological engineers.
- With the 'Big Pharma' method of developing drugs, it takes 10-15 years for a scientific discovery to result in improved patient care [8][9] (some authorities even say 20 years [10]). In other words, now in 2012, patients receive the best medical technology 1997 has to offer. In an era of such rapid scientific discovery, this is simply not good enough. It is imperative that society develop a quicker pipeline between scientific discovery and clinical care, to allow sick people to benefit from the recent advances in medical science. Open source medical research is this pipeline.
Emerging treatments
Regenerative medicine
Regenerative medicine is an emerging science which aims to grow new body parts. These could either be grown in the lab and transplanted into the patient's body, or else the patient's body could be coaxed into regrowing damaged or missing parts. Your cellular machinery and genetic code was able to grow two legs already - why shouldn't it be able to grow another if you lose one in an accident? Mammalian embryos can regrow a limb if it is cut off in the first trimester of pregnancy; this is a native ability of the body, but when we age, something shuts this ability down. If we understand what this something is, we will be able to turn it off and restore the body's ability to grow organs and limbs. This will allow people suffering organ failure to have replacement organs on demand. Because their own DNA is used, their body will not attack it as foreign, as happens with transplants. Amputees could regrow their own limbs instead of using prostheses. People with spinal cord injuries could grow back their damaged nerves.
Though far from complete, regenerative medicine in progressing in leaps and bounds. The key to regenerative medicine is stem cells, undifferentiated cells that can become any kind of cell. A series of breakthroughs from 2007-2009 made it possible to take adult cells and turn them into stem cells, known as Induced pluripotent stem cells . These stem cells are the starting point for culturing new organs. Trials are currently underway in using induced pluripotent stem cells to regrow damaged spinal nerves.
3D printing technology, using desktop printers with cells in the cartridges instead of ink, can lay down different kinds of cells in precisely-controlled patterns and build human organs. See this video for the current generation of technology. Scaffolds made of biomaterials such as collagen can be used to provide a structure onto which the cells are then applied.
Similar methods applied to animal muscle could provide a less resource-intensive way of growing meat for food - see In-vitro meat.
Robotic surgery

One key advantage of robotic surgery is that the movements of the surgeon's hands can be miniaturized; a surgeon can make a movement and the robotic hand can emulate the same movement, but 100 times smaller. Robotic surgery also eliminates the tiny tremors that surgeons train so hard to control, but which are an inevitable part of all fine human movement. Future generations of surgical robots will miniaturize this further, down to the micrometer level. Such robots could then even be inserted into the patient and controlled remotely from the outside. As Richard Feynman quipped in his original proposal of nanotechnology, "It would be interesting in surgery if you could swallow the surgeon."
Recent progress in the areas of human-machine interface will also lead to more intuitive and precise surgical robots. For instance, a Microsoft Kinect has been modified to perform surgery [11]. The advanced scanning methods mentioned above, combined with augmented reality displays, can even be used to allow the surgeon to see inside the patient before making an incision.
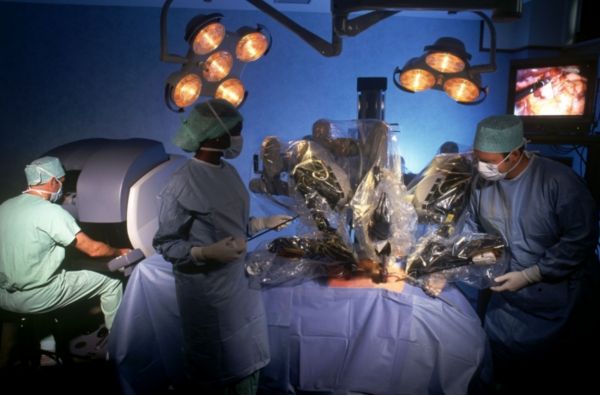
Nanomedicine
The future may see very complex nanotechnology devices, the size of cells, that can go into the body and perform all sorts of complex tasks. See http://www.foresight.org/nanomedicine
Somatic gene therapy
Much research is being done into somatic gene therapy: the attempt to change the DNA of an adult by inserting specific genes using vectors. None have yet been successful in humans, though it is common practice in mice. This could cure all genetic diseases.
The fight against cancer
Early detection and prevention
As discussed above, information-rich medicine is preventive and tends to pick up diseases very early by using always-on sensors and recognizing subtle patterns. This is never more important than with cancer, where early detection makes all the difference.
Researchers have built up a good idea of the environmental, genetic and other risk factors that put a person at high risk of cancer - but it is not the researchers who need to know, it is the person himself! Open-source, information-rich medicine can alert people at high risk of cancer (or, indeed, any disease), and advise them on preventive measures. For example, if your Electronic Medical Record shows you have lived near a chemical processing plant that increases risk of cancer, and you have family history of cancer, and you have a gene that is correlated with high rates of cancer, the A.I. could alert you by text message, and give you reminders to consume more anti-angiogenic foods and 'medicinal' mushrooms. When genetic screening becomes cheap enough to be commonplace, this personalized, preventive approach will be very effective. Cancer is basically a preventable disease[12], and people are more likely to comply with messages that are tailored to them than to broadcast public health campaigns, so using this method, a large fraction of cancer cases should be prevented.
Proteomics
Where cancer cannot be prevented, A.I., empowered by proteomic data, will soon be able to design systemic interventions that disrupt the molecular causes of the cancer. Rather than give people chemotherapy and hope it works, proteomic analysis will allow for a computer simulation to be built to show exactly what is happening with your biochemistry, and to simulate the effects of various interventions. These interventions may not necessarily attack the tumor itself, or even try to shrink it; as discussed above, A.I. can design interventions that work by altering the balance of biochemical system, so some of the interventions may appear unrelated to cancer; they may be such things as giving a drug that builds bone mass[13], or altering amino acid ratios in your diet.
New weapons against tumors
Attacking the tumor will still remain a useful tactic, though, and we have several new and sophisticated weapons. Robotic surgery is one.
Another is a kind of nanostructure known as a dendrimer, displayed to the right. Dendrimers are intricate nanoengineered molecules with beautiful snowflake-like structures. Different molecules can be attached to the surface of the structure, allowing dendrimers to act as highly customizable drug-delivery vehicles. For example, folic acid can be attached at one point on the dendrimer and an anti-cancer agent attached at another point. Because cancer cells have a hunger for folic acid, the dendrimer will be absorbed by the cancer cells, where the anti-cancer drug will kill them. This allows far lower doses to be used than if the anti-cancer agent were used alone - and more importantly, it targets only the tumor, resulting in much less toxicity to non-cancerous cells[14][15] Dendrimers can also be designed to go to the tumor site and release a florescent protein, allowing a surgeon (perhaps robot-assisted) to see exactly what tissue is and is not cancerous.A third new and elegant weapon to destroy tumors is with photodynamic therapy . This involves using compounds that are benign in themselves, but when stimulated with burst of light, release radical compounds that destroy all tissue around them. A patient is given these drugs, and then a burst of light is delivered directly onto their tumor by fiberoptic cable.
Tumor-treating fields are a new modality for stopping the proliferation of cancer cells. Electrodes generate electric fields tuned to the proteins that cancer cells use to divide. The force generated on the proteins inhibits their normal movement and the cells cannot divide properly. This is a very safe treatment and free of side-effects, though it is somewhat slow to work.
A similar method is Irreversible Elecroporation, which punctures the membranes of cancerous cells using high-energy electric fields, leaving healthy cells untouched.